Introduction
The spatial resolution of any imaging modality based on waves (electro-magnetic or mechanical) is fundamentally limited by the size of its wavelength (diffraction-limited image resolution). In biomedical ultrasound imaging this diffraction limit has resulted in an inherent compromise between spatial resolution and penetration depth. Recently such diffraction limit has been broken in the field of optical microscopy, by the so-called “super-resolved fluorescence microscopy” which was awarded the 2014 Novel Prize in Chemistry.
We are working on the ultrasound counterpart of this super resolution imaging, by developing techniques to detect and pin-point individual microbubble contrast agents within micro-vasculature. We have generated both in vitro and in vivo images of image resolution far below the diffraction limit [1, 2]. The figure below shows the first in-vivo super-resolved images of a mouse hear.
We are working with a number of collaborators to further improve the technique and apply this to a wide range of in vivo applications.
Please find below some further information on our technique and some current projects.

Figure 1 – First in-vivo super-resolution images of a mouse hear. (a) Localisation Map. (b) Angle Map. (c) Velocity Map.
Super-Resolution Processing Framework
We have developed a processing framework to localize and track high-concentration microbubbles at low frame rates, alleviating super-resolution imaging’s reliance on ultrafast ultrasound systems and long acquisition times that are often not available clinically. We have applied this framework to various imaging targets with different ultrasound systems. The effectiveness of the framework was validated by imaging animal brains using down sampled frame rates and human lymph nodes using data acquired by commercial systems in routine clinical management protocols.
The figures below show two recently reconstructed SR images of a human lymph node (left) and a rat brain (right) are shown below. Technical details can be found in [3]. We are optimizing beamforming/reconstruction methods for 3D SR imaging and developing an easy-to-use interface for clinicians.

Figure 2 – Super-resolution images of human lymph node (left) and a rat brain (right)
Acoustic Wave Sparsely-Activated Localization Microscopy (AWSALM)
Ultrasound Localization Microscopy (ULM) provides very high spatiotemporal resolution, but the use of microbubbles requires low contrast agent concentrations, a long acquisition time, and gives little control over the spatial and temporal distribution of the microbubbles. Acoustic Wave Sparsely-Activated Localization Microscopy (AWSALM) and fast-AWSALM for in vivo super-resolution ultrasound imaging, offering contrast on demand and vascular selectivity with the use of nanodroplets. We have demonstrated the feasibility of fast and selective measurement of microvascular dynamics in vivo with subwavelength resolution using ultrasound and acoustically activatable nanodroplet contrast agents [4].

Figure 3 – The deliberate activation of nanodroplets allows for spatially selective super-resolution imaging in vivo.
Broad Elevation Projection (BEP)
We proposed a Broad Elevation Projection (BEP) SR imaging method using an unfocused 1D array probe that has a similar fabrication and computational cost as conventional 2D SR imaging, and similar volume coverage as full 3DSR imaging but with an order of magnitude less fabrication and computational cost [5].

Figure 4 – In vivo localization, velocity amplitude and velocity angle maps of the conventional 2D, BEP and MIP of the 3D SR imaging methods.
Coronary Ultrasound Localization Microscopy
ULM can measure parameters such as vascular tortuosity and blood flow with unprecedented detail and accuracy. A limitation of 2D ULM is out of plane flow, complex three-dimensional vasculature and motion. This limits the ability of ULM to measure myocardial microvasculature structures. We demonstrate the feasibility of deep 3D ULM with a matrix array in a large porcine model with realistic motion to narrow the translational gap between research and meaningful clinical applications [6].

Figure 5 – Microvasculature of the myocardium in an ex vivo porcine heart.
Non-invasive detection of lymph node metastasis
Currently the best way to find cancerous lymph nodes related to breast cancer is to surgically remove them and look for metastases. Armpit surgery is necessary because there are no radiology tests reliable enough to replace the operation. The aim of this research is to develop a new ultrasound test to replace armpit surgery.
The figures below show a lymph node following an intravenous injection of ultrasound contrast agent. The videos highlight the contrast circulation in the blood system and their corresponding maximum intensity projection (accumulation) after few seconds of acquisition. Localisation and tracking of the contrast is provided and then investigated to identified benign and malignant lymph nodes.
Figure 6 – Intravenous flow visualization of ultrasound contrast agent in an armpit lymph node and its corresponding microvasculature.
Super-resolution imaging of myocardial microvasculature
Cardiac microvasculature plays an important role in cardiac myocardium perfusion and microcirculation. Limited by the spatial resolution of the current imaging techniques, the anatomical assessment of the microcirculation is challenging. This project aims to use the super-resolution ultrasound imaging technique to visualise the microvasculature in the cardiac myocardium.

Figure 7 – A: Super resolution myocardial microvasculature map for a Langendorff ex-vivo pig heart, short axis view. B: Flow direction map for the zoomed-in region inside the rectangular box of Figure A. Angle map is showed right to the figure B. C: Flow velocity map for the same zoomed-in region. Velocity map is showed right to the figure C. LV: left ventricle; RV: right ventricle.
Super-resolution imaging of the gut microvasculature
The gut has been one of the less studied organs of the body and people are starting to realise how important is the role it plays in our body. Today, the gut micro-vascularisation is very little known but understanding it could bring us a lot of information on the gut functioning and its diseases. Therefore, this project focuses on the analysis of the gut microcirculation in response to food and therapeutics intakes.

Figure 8 – Angle map and velocity map of a gut loop of a rat small intestine.
Monitoring the breast tumour microenvironment following radiotherapy using super-resolution ultrasound and functional MRI
This project aims to develop robust quantitative parameters to detect biological changes before tumour size changes, in the hope of identifying response, resistance or relapse earlier than is currently possible.
The figures below show normal breast microvasculature and the breast tumour microvasculature before treatment. The tumour microvasculature is seen to be more densely vascularized and chaotic. The breast tumour microvasculature 12 months post radiotherapy treatment visually appears to have normalised.
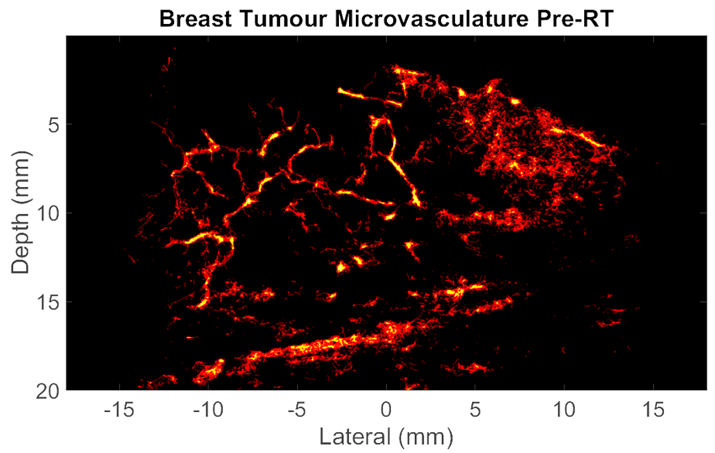
Figure 9 – Normal breast microvasculature, the breast tumour microvasculature before treatment and 12 months post radiotherapy treatment
References
[1] Christensen-Jeffries, K., et al., In vivo acoustic super-resolution and super-resolved velocity mapping using microbubbles, IEEE Trans Med Imaging, 2015, 34(2), p.433-40.
[2] Viessmann, O.M., et al., Acoustic super-resolution with ultrasound and microbubbles, Phys Med Bio, 2013, 58(18), p.6447-58.
[3] Yan J., et al. “Super-Resolution Ultrasound Through Sparsity-Based Deconvolution and Multi-Feature Tracking,” IEEE Transactions on Medical Imaging, vol. 41, no. 8, pp. 1938-1947, Aug. 2022
[4] Kai Riemer, Matthieu Toulemonde, Jipeng Yan, Marcelo Lerendegui, Eleanor Stride, Peter D Weinberg, Christopher Dunsby, Meng-Xing Tang, Fast and selective super-resolution ultrasound in vivo with acoustically activated nanodroplets, IEEE Transactions on Medical Imaging, 2022 Nov 18; PP, doi: 10.1109/TMI.2022.3223554.
[5] Bingxue Wang, paper link: https://arxiv.org/pdf/2206.03912.pdf
[6] Kai Riemer, Konstantinos Ntagiantas, Biao Huang, Matthieu Toulemonde, Jipeng Yan, Justin Perkins3, Christopher Dunsby, Rasheda A Chowdhury, Meng-Xing Tang, 3D super-resolution ultrasound imaging of deep cardiac microvasculature in an ex vivo porcine heart, 28th European Symposium on Contrast Ultrasound Imaging.